The last decade has witnessed significant investment in the research, development and deployment of 5G networks and systems throughout the world. The deployment of 5G systems is currently ongoing and it is rightfully expected that the addition of new communication services and capabilities will inevitably be reflected in the way we operate as society. The changes brought about by the advances in 5G are expected to play a transformative societal role, with demands placed upon the technology eventually and ultimately becoming greater than the technology can deliver. The interplay between the evolving societal needs and the push from the advancing technological tools are expected to play a major role in the definition of the new generation of communications – 6G [1].
Major technological advances in the areas of hardware, cloud, open source, continuous evolution of DevOps, AI and Internet evolution are expected to play a pivotal role in the rise of new services and capabilities the future networks will need to provide. Even though the exact specifications to be imposed on the new generation of networks are difficult to predict with certainty, the evolution path of 4G towards 5G provides a hint of possible directions. It will, therefore, not come as a surprise that future networks will be expected to cater for an exponentially increasing traffic, stemming from a variety of scenarios, with examples covering healthcare, cyber, AI and immersive communication platforms, to name but a few. These platforms will not only necessitate extremely high data rates, network resilience and adaptability, but they will also be expected to provide a sub-millisecond latency in the end-to-end scenario and be able to avail of flexible spectrum usage [2].
Flexible spectrum usage refers to the ability of communications devices to adapt its RF front end in accordance with the needs and requirements of the communications system. Here, adaptation refers to the capability of communications hardware (RF and mm-wave) to adapt itself in terms of the operating frequency, bandwidth and radiation characteristics, so as to support the new applications and services of the forthcoming 6G standards. It is believed that the lower frequency bands (below 6 GHz) will remain of importance to 6G, as much as they are to current 4G/5G technologies, however, it is also expected that the mm-wave spectra (24 GHz-52 GHz) already used by 5G will also be extended to 100 GHz so that it could be used for 6G. There are expectations that the spectrum above 100 GHz [3] will also need to be utilised, however, major technological advances are necessary to address the problem of challenging propagation environments.
To address the above-mentioned future challenge related to hardware for 6G, major technological advances on the level of new materials, RF and mm-wave devices and Transceiver (TRX) architectures are needed. The hardware research challenge in 6G is therefore three-fold:
- New RF and mm-wave materials enabling tunability and re-configurability.
- New RF and mm-wave devices capable of exploiting the capabilities of new materials.
- New TRX architectures capable of exploiting the capabilities of new materials and new devices.
As indicated earlier, new services and new applications will require a great deal of flexibility, to the extent that such flexibility is impossible to cater for using the existing reconfigurable technologies. In other words, solving the problems of the future, requires the tools of the future. Such new materials are expected to act as the fuel for the development of new devices, with new capabilities, and will be able to support new transceiver architectures needed for flexible spectrum usage.
State-of-the-art & Limitations
Current reconfigurable RF and mm-wave hardware is still in infancy. Even though re-configurability has been present in RF devices and components for at least two decades, these devices have traditionally experienced high levels of insertion losses and low switching speeds and, have almost always been an afterthought [4-7]. These early tuneable devices usually included the addition of a semiconductor device (diode or transistor) to a standard, passive RF device (filter, antenna, phase shifter) in order to gain a degree of controllability, to a limited extent. There have been, however, several efforts to integrate externally controllable bulk tuneable materials, such as Liquid Crystals (LCs) [8-10], Ferro-Electrics (FE) [11-13] and, most recently, Electro-Chromic (EC) materials [14-17] with RF and mm-wave circuits, such as filters, antennas and phase shifters. However, as mentioned earlier, such approaches have not yielded a great deal of commercial traction, since the electrical and size requirements imposed on commercially available RF and mm-wave hardware are very strict and, simply cannot be met using the reconfigurable technologies available today. For example, the loss tangents of LC mixtures still tend to be quite large – of the order of 0.03 [9], which is considered too large for many ap-plications, while their response time usually of the order of a few ms. Ferro-electrics, even though offering larger tuneable ranges than LCs exhibit very high dielectric constants which are of limited use for RF and mm-wave applications. The research on EC materials is, however, still in infancy, however it appears to offer a compromise between the LCs and Fes, with an added benefit of exhibiting a strong memory effect, [18-21]. The devices and architectures that these traditional bulk-tuneable materials can support, in the context of future networks, is, therefore, limited to, for example, phase shifters, attenuators, to name but a few, where the integration with semiconductor technologies is easier.
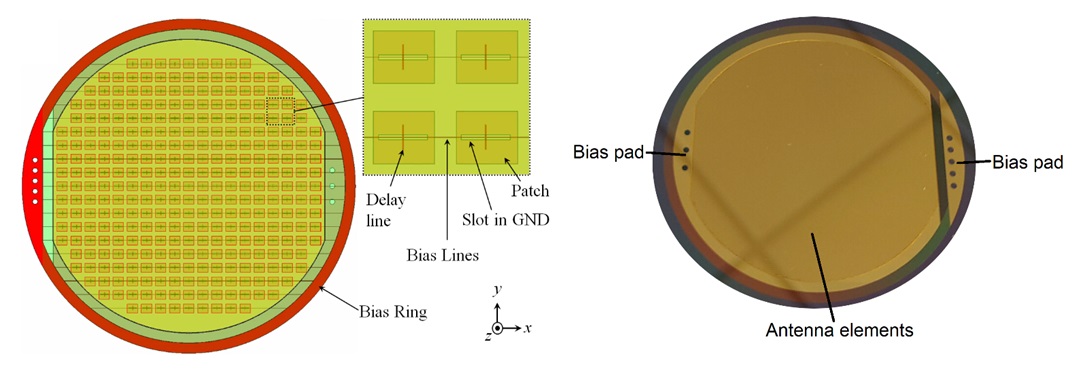
Increased re-configurability is expected to benefit the upcoming 6G communications in a paradigm-shift way. An example of these are Intelligent Reflecting Surfaces (IRS), which have gained an increased degree of in-terest lately. IRS have recently attracted a great deal of attention, especially since they allow the attainment of some form of re-configurability, which is of great importance in the context of 5G and the upcoming 6G specifications. In particular, IRS have been used to mitigate the harmful effects of the wireless environment by virtue and ability to redirect the incoming signal towards a specific path. This ability is usually achieved by controlling some parameters of meta-atoms, such as the phases and amplitudes. The elements of IRS can be controlled through either a semi-conductor device, Micro-Electro-Mechanical Switch (MEMS) or liquid crystals [22], depending on the parameter of the meta-atoms that is being controlled. In turn, this allows the IRS to manipulate the incident wavefront to achieve beam-steering, adjustable absorption, polarisation, filtering and collimation [23]. However, the losses and latency times of the constituent materials limit their application range, [24].
In a similar vein, frequency tunability/re-configurability constituent materials is greatly expected to enable entire novel RF and mm-wave front ends. For example, traditional RF and mm-wave front ends, such as beam-former networks, still rely on semiconductor components to achieve beam steering, where filter banks are, still, passive and not tunable/reconfigurable. However, recently there has been a rise in the “re-invention” of some of the older antenna technologies, such the Luneburg and Rotman lenses [25-29]. The interest in such technologies has been fuelled by the availability of advanced manufacturing techniques (3D plastic and metal printing), but also by the fact that new communications frequencies are expected to be in the mm-wave region, which significantly reduces their physical size. Nevertheless, introducing re-configurability/tenability into these structures is, from the standpoint view of current technologies, a severe limitation.
Novel Approach
Multifunctional materials, i.e. materials that can adapt to the external environment will be the key for future devices. 2D materials hold a unique position in the design of such structures. The family of 2D crystals these days hold a number of different one-atom-thick materials: insulators, semiconductors, metals, thus giving rise to very complex van der Waals heterostructures where such 2D crystals can be combined together to form artificial materials with predetermined properties. Furthermore, combining such crystal with other materials, for instance polyelectrolytes, it is possible to make them responsive to the environment and to force them to adapt to external conditions.
In order to address the 6G communications challenge in an appropriate manner, it is imperative for RF practi-tioners to interactively engage with material scientists in order to create new functional RF and mm-wave materials that will fuel the development of agile RF and mm-wave hardware. Here, we would like to mention Transition Metal Oxides, Phase Change Materials and other bespoke 2D materials, capable of high dynamic ratios and high switching speeds that have the capacity to fuel the development of future hardware, such as:
- Reconfigurable filters. Filters are not only expected to be the direct beneficiaries of the advances in new materials but based on the high switching speeds of such new materials, a new paradigm shift in filtering architectures is expected. Examples are filter configurations obtained using high-speed switches, where the shape of the passband can be tailored by weighted sampling.
- Reconfigurable antennas. Antennas are an additional beneficiary of the advances in new materials. Here it is envisaged that such switches can be used in the circuit of meta-material lens antennas in order to create variable radiation patterns.
- Intelligent surfaces (IS). It was mentioned earlier that in the current state the insertion losses and la-tency of IRSs preclude their widespread use. For example, the best-in-class insertion losses of a state-of-the-art semiconductor switch is about 0.15 dB up to frequencies of 6 GHz. IRSs are, in gen-eral, composed of hundreds, if not thousands, of such switches, which, inevitably increases the total insertion losses. In this aspect a combination of the new materials/switches and innovative RF archi-tectures can be used to create low-loss IRS.
- THz communications. The interest in THz communications has been growing in recent years, driven, primarily, by the expectations that future communications will be able to take advantage of advanc-ing technological tools. This has been followed closely by the resurgent interest in some technologies developed in the 1950s, such as the Resonant Tunnelling Diode (RTD) [30 – 34]. Since the RTD, in addi-tion to exhibiting very high switching speeds and having a region of negative dynamic resistance, makes the prospect of research and development of novel transceiver architectures capable of ex-ploiting this phenomenon very attractive. This has the potential to give rise to new transceiver archi-tectures, such as novel THz beamforming technologies, where the negative dynamic resistance can be employed to create radiating structures and how such structures could be combined in a variety of topologies, such as a beam-forming network, or smart THz surface.
The creation of reconfigurable/ “smart” RF materials will increase the need for “smart” control of RF and mm-wave hardware. Here, Artificial Intelligence (AI) will be expected to play a crucial role.
References
[1] https://www.ericsson.com/en/reports-and-papers/white-papers/a-research-outlook-towards-6g
[2] https://www.controleng.com/articles/important-technological-developments-to-watch-for-6g/#:~:text=In%206G%2C%20the%20frequency%20ranges,(0.3%20to%2010%20THz)
[3] https://www.ericsson.com/en/reports-and-papers/ericsson-technology-review/articles/the-future-of-cloud-computing
[4] A. Malczewski et al., ‘X-band RF MEMS phase shifters for phased array applications’, IEEE Microwave and Guided Letters, vol. 9, no. 12, pp. 517-519, December 1999.
[5] H. T. Kim et al., ‘A compact V-band 2-bit reflection-type MEMS phase shifter’, IEEE Microwave and Wireless Components Letters, vol. 12, no. 9, pp. 324-326, September 2002.
[6] C. L. Chen et al., ‘A low loss Ku-band monolithic analog phase shifter’, IEEE Trans. Microwave Theory Tech., vol. MTT-35, no.3., pp. 315-320, March 1987.
[7] D. M. Krafcsik et al., ‘A dual varactor analog phase shifter operating at 6– 18 GHz’, IEEE Trans. Microwave Theory and Tech., vol. 36, no.12, pp.1938-1941, December 1988.
[8] R. James, F. A. Fernandez, S. E. Day, S. Bulja and D. Mirshekar-Syahkal, “Accurate modelling for wideband characterisation of nematic liquid crystals for microwave applications”, in IEEE Microwave Theory and Techniques, pp. 3293-3297, vol. 57, issue 12, 2009.
[9] S. Bulja, D. Mirshekar-Syahkal, R. James, S. E. Day and F. A. Fernandez, “Measurement of dielectric properties of nematic liquid crystals at millimetre wavelength”, in IEEE Microwave Theory and Techniques, pp. 3493-3501, vol. 58, issue 12, 2010.
[10] M. Yazdanpanahi, S. Bulja, D. Mirshekar-Syahkal, R. James, S. E. Day and F. A. Fernandez, “Measurement of dielectric constants of nematic liquid crystals at mm-wave frequencies using patch resonator”, in IEEE Transactions on Instrumentation and Measurement, pp. 3079-3085, vol. 59, issue 12, 2010.
[11] R. R. Romanofsky, “Advances in scanning reflectarray antennas based on ferroelectric thin-film phase shifters for deep space communications”, in Proceedings of the IEEE, pp. 1968-1975, vol. 95, issue 10, 2007.
[12] M. Haghzadeh, C. Armiento and A. Akyurtlu, “All-printed flexible microwave varactors and phase shifters based on a tunable BST/Polymer”, in IEEE Microwave Theory and Techniques, pp. 2030-2042, vol. 65, issue 6, 2017.
Leave a Reply