There has been a great deal of talk about 6G, but it is not very clear what exactly 6G is supposed to be. Simply, there are no standards, as of now, that clearly spell out the benefits that 6G communications standards will bring and what gaps in the current 5G communication standards the new 6G communication standard will address. That is not to say that academic literature is scant of ideas as to what 6G should be like, however, most of those fail to paint a bigger picture regarding what exactly 6G is going to be and why do we need it? Some good hints could be found in a variety of white papers, such as those from Ericsson [1], which clearly spell out that 6G will not only be driven by a technological push, but also the societal pull. Let us go briefly through the generational journey of how we ended up where we are to shed some light on what 6G may actually look like. We will start with the 4G communications standard, as going further in the past will not bring useful insights.
The 4G communications standard offered a great deal of improvements compared to the previous generation (3G) communication standard, namely speed, latency and capacity. In terms of speed 4G networks could deliver download speeds up to 150 Mb/s and upload speeds of 50 Mb/s, which was a marked improvement compared to the 3G equivalents – 7.2 Mb/s and 2 Mb/s, respectively [2]. Latency differences were also much more favorable for the 4G standards, averaging between 50 ms and 80 ms, compared to the 3G networks where the average stood between 60 ms and 340 ms [3]. However, from the technical point of view, the most marked difference was the use of advanced antenna techniques – such as beamforming and Massive Input Massive Output (MIMO). In this aspect, Alcatel-lucent introduced the concept of lightradio [4], a beamforming technique, which relied on the use of individual “cubes”, with each cube containing a standalone transceiver and antenna. The greater the number of “cubes”, the greater the capability to perform beamforming and hence, capacity of the system. In terms of operational frequencies – both the 3G and 4G standards operate at frequencies below 3 GHz, which ensured wide coverage at the expense of limited achievable data rates.
The need for greater speeds, lower latencies and capacities gave rise to 5G. As an example – 5G offers peak data dates up to 20 Gbps and latencies between 8 ms-12 ms, which is a tremendous improvement compared to 4G. However, it is important to distinguish between 2 flavors of 5G – Future Radio (FR)1 and Future Radio (FR) 2. FR 1 is exemplified using frequencies below 6 GHz and, in terms of capacity and speeds, represents only a minor improvement compared to 4G. However, the strength of 5G comes to light in FR 2, which occupies frequencies from 24 GHz to 71 GHz, with the frequency spectrum between 24.25-29.5 GHz [5] being the most used 5G mm-wave spectrum range. 5G FR 2 (or mm-wave 5G) indeed offers impressive data rates, albeit only for a limited spatial range. This is primarily since free space attenuation at mm-wave frequencies, i.e., the frequencies of 5G FR2 is a magnitude higher compared to the losses at the frequencies of FR 1 (below 6 GHz). In addition to enabling unprecedented data rates compared to 4G, 5G is also poised to aid the proliferation of Internet of Things (IoT) devices, in particular at mm-wave frequencies where the size of the RF front end (filters and antennas) is much smaller compared to their size at frequencies below 6 GHz.
Given this brief overview of 4G and 5G communications standards, one may rightfully ask – why 6G? What are the benefits? What will 6G be capable of that 5G is not? Simply put, this is not fully known at this stage, but as the white paper from Ericsson states, it is expected to be a combination of technological push and societal pull. In other words, the drive towards 6G will be human-centric. The frequencies of 6G communications standards are not known at this stage, however, it is strongly believed that 6G frequencies will extend to over 100 GHz [1]. To be exact, the frequency range under consideration for 6G includes W-band (75 GHz-110 GHz), D-band (110 GHz – 175 GHz) and in some instances even higher frequency bands.
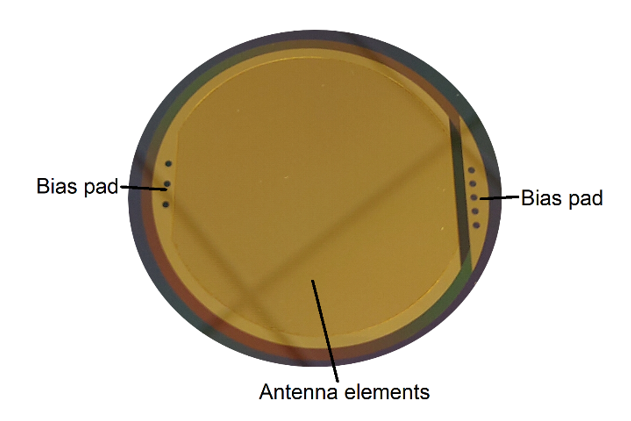
are mentioned (275 GHz – 300 GHz, and from 0.3 THz – 10 THz) [6]. Given the lessons that we have learnt from 5G FR 2, increasing the frequency of operation ultimately reduces the spatial range due to increase in the losses, infers that 6G networks are inherently poised to cater for low spatial ranges with extremely high data rates. This, in turn, suggests that networks of the future will be highly dense with a variety of micro, pico and femto cells overlapping each other to provide good coverage. This may work well for urban environments, but in rural environments it would impractical and cost ineffective. However, that is not the main problem.
One of the biggest technical problems that 6G communication networks face lies with the need to address backword compatibility, as such new networks will be expected to perform well in the context of 5G and possibly 4G, which, as shown earlier, operate at lower frequencies compared to prospective 6G. In other words, 6G networks, in addition to catering for extremely high data rates, being highly resilient and of low latency, will also need to be able to avail of flexible spectrum usage [7], inferring that they must be adaptable to receive and transmit signals of a variety of frequencies. As we have seen, such frequencies can range from below 6 GHz (5G FR1), through mm-wave frequencies (24 GHz – 71 GHz, 5G FR2) up to, possibly, 300 GHz. From the Radio Frequency (RF) hardware point of view, this is a tremendous, if not impossible, task to accomplish which can only be achieved using reconfigurable hardware – the “holy grail” for RF stalwarts. This is the problem. Reconfigurable technologies enabling hardware adaptability, even though, subject to academic and industrial research for over 3 decades have not yield much traction – they are either too lossy and slow such as the case of Liquid Crystals (LCs) [8], non-conducive to RF – FerroElectric (FE) [9] or difficult to integrate into RF circuits, such as semiconductors. Solving this problem or, at least, reducing the impact of this problem will be of tremendous importance to paving the way to 6G. At present, however, there is no obvious way how this will be achieved. However, if and when solved, it would allow a plethora of novel types of RF communication devices, such as Intelligent Surfaces to be fully coupled with the advances made with respect to Artificial Intelligence (AI) to reach a real-world impact that be of benefit to everyone. Until then, research continues.
References:
[1] https://www.ericsson.com/en/reports-and-papers/white-papers/a-research-outlook-towards-6g
[2] https://en.wikipedia.org/wiki/4G
[4] https://money.cnn.com/2011/03/21/technology/light_radio/index.htm
[5] frequency spectrum between 24.25-29.5 GHz being the most used 5G mm-wave spectrum range
[8] S. Bulja, D. Mirshekar-Syahkal, R. James, S. E. Day and F. A. Fernandez, “Measurement of dielectric properties of nematic liquid crystals at millimetre wavelength”, in IEEE Microwave Theory and Techniques, pp. 3493-3501, vol. 58, issue 12, 2010.
[9] M. Haghzadeh, C. Armiento and A. Akyurtlu, “All-printed flexible microwave varactors and phase shifters based on a tunable BST/Polymer”, in IEEE Microwave Theory and Techniques, pp. 2030-2042, vol. 65, issue 6, 2017.
Leave a Reply